Many types of cancer, chemotherapy, and numerous other underlying conditions can leave patients vulnerable to infection by bacteria that would normally be unable to survive in the body. These opportunistic infections are challenging to treat, as antibiotics are often ineffective against these pathogens or have serious side effects not well-tolerated by individuals with underlying conditions. Dr. Sullivan studies how opportunistic infections occur, with a particular focus on lung infection. His ongoing work is centered on identifying bacterial adaptations that enable pathogens to survive both the host defense systems present in the lung as well as antibiotic treatment. His work will help deepen our understanding of opportunistic infection and may provide novel avenues for more effective and tolerable treatment of these pathogens.
All Cancers
Current Projects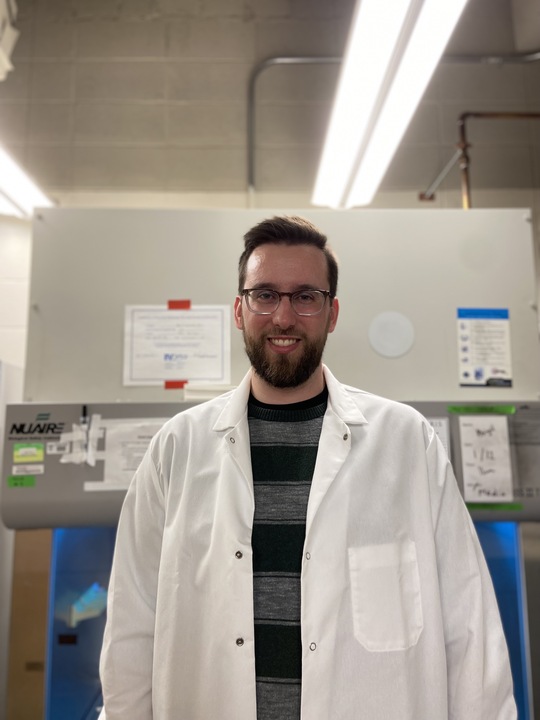
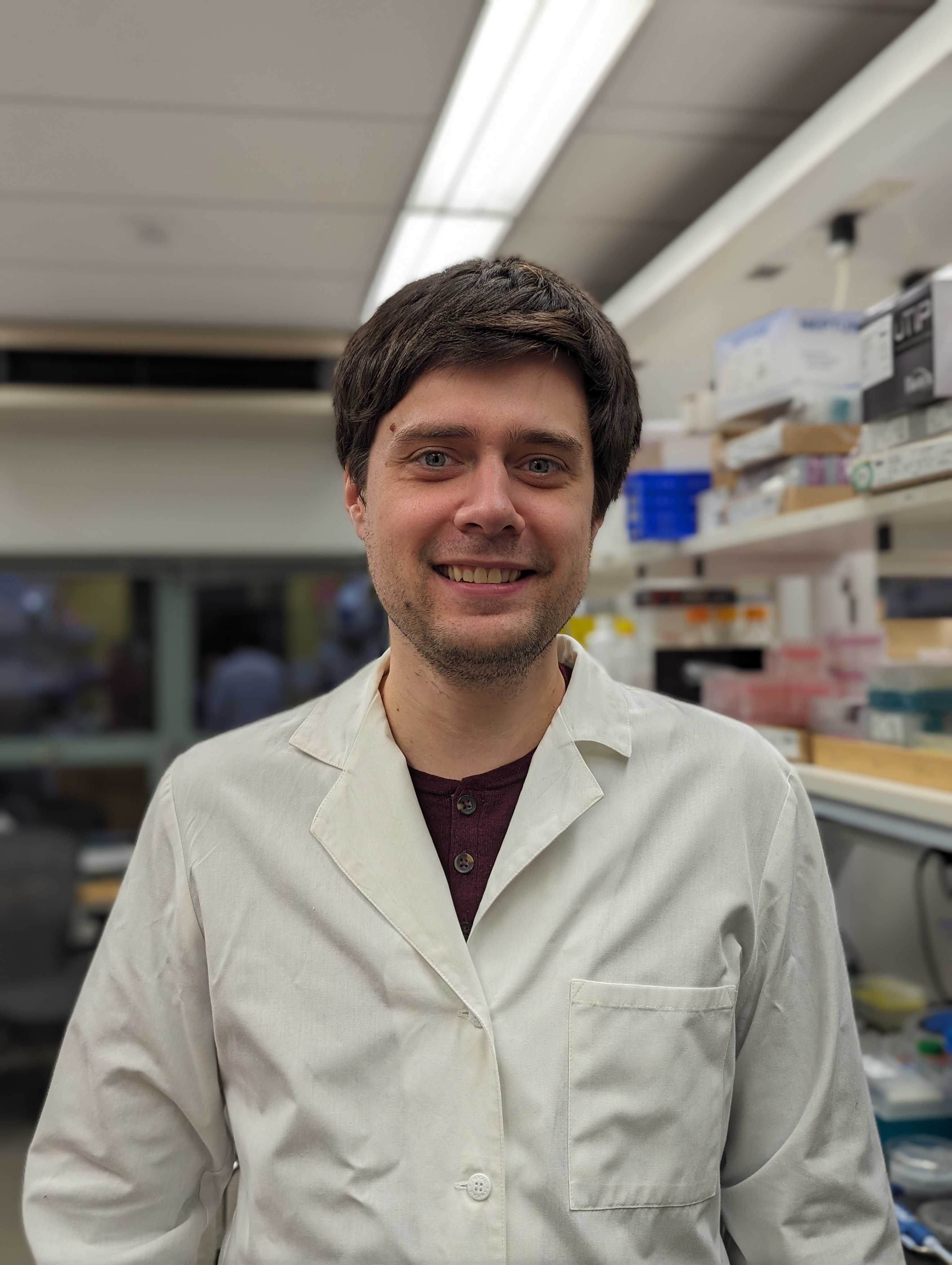
Antimicrobial resistance is a growing crisis that imperils our ability to protect patients immunocompromised by cancer treatment. Despite this, the few new antibiotics currently in clinical trials primarily use established mechanisms of action. Identification of new targets for antimicrobial drugs is thus an urgent clinical need. Recent work has shown that bacteria can tolerate substantial inhibition of many proteins thought to be essential for growth, rendering them poor drug targets. The mechanisms that cause this robustness are poorly understood. By combining cutting-edge microfluidic technologies with methods for controlled gene repression, Dr. Taggart will systematically identify mechanisms that allow bacterial cells to tolerate inhibition of genes critical for cellular growth. This work will guide the selection of targets for future antibiotic development and may reveal mechanisms by which to sensitize bacterial cells to existing drugs. Dr. Taggart received his PhD from Massachusetts Institute of Technology, Cambridge and his BS from Haverford College, Haverford.
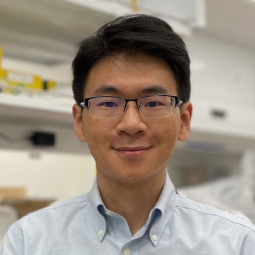
Dr. Tai studies bacterial biofilms or aggregates of bacterial cells in an extracellular matrix. Biofilms play a critical role in many health and industry settings. Biofilm-forming bacteria and imbalance in patients’ gut microbiota have been found to correlate with cancer development, and cancer patients receiving therapy frequently suffer from bacterial infections. From the unique perspectives of microbiology, soft matter physics, and ecology, Dr. Tai aims to decipher how, at the single bacteria cell level, heterogeneities in cell shape, organization, and gene expression constitute the function and development of their collective communities: biofilms. His work is expected to deepen our understanding of bacterial biofilms and ultimately contribute to therapeutic strategies.
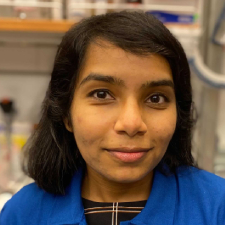
Dr. Thawani [Merck Fellow] studies selfish DNA sequences—so called because they copy and paste themselves within the human genome despite offering no specific fitness advantage. Dr. Thawani will utilize advanced methods such as cryo-electron microscopy to reveal the cellular machinery that assists these selfish elements and thus delineate their mechanism of mobility. She will use this insight to engineer new genome editing technologies to precisely insert large genes at user-specified sites in a variety of human cell types. This general technology will not only translate directly into new gene therapies, but also result in wide-ranging applications in synthetic biology. Ultimately, this work will contribute to treatment for many cancer types, including improved CAR-T therapies for blood cancers.
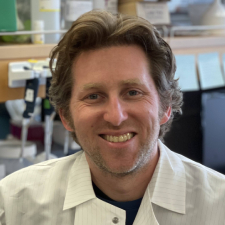
Chimeric antigen receptor (CAR) T cells are immune cells that have been genetically engineered to bind specific proteins on cancer cells. CARs can display exquisite sensitivity and discrimination, and CAR T cells have been deployed with spectacular success to detect and kill blood cancers. Unfortunately, they are much less effective against “solid” tumors, such as breast or kidney cancers. To address this problem, Dr. Titus [Connie and Bob Lurie Fellow] is designing T cells with membrane proteins that perform novel functions, including proteins that facilitate membrane fusion or alter the adhesion between T cells and their targets. By redesigning T cell membranes, Dr. Titus hopes to create useful cancer-fighting tools that can be deployed in conjunction with other emerging cellular therapies and immunotherapies. Dr. Titus received his MD and PhD from the University of California, San Francisco, and his AB from Harvard University.
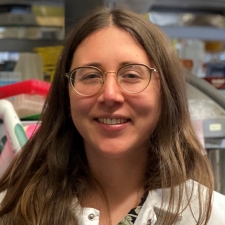
When an organism is developing, it must correct mistakes that might occur at the level of individual cells or tissues. Dr. Triandafillou [National Mah Jongg League Fellow] wants to better understand how error correction systems work, and why they might not work in cases like cancer. To explore these developmental questions, Dr. Triandafillou uses what are called gastruloids, 3D clusters of stem cells that can organize themselves and transform into the basic building blocks of an organism. She developed a method using microscopy to trace the history of these cells and measure how much their past state and history influence what they become. Dr. Triandafillou wants to see how differences in individual cells might impact what those cells eventually turn into, and how such differences affect the correction of mistakes like abnormal growth, bias in cell types, or missing cell types. She is also interested in how the cells around an error react to it. Dr. Triandafillou received her PhD from the University of Chicago and her BS from Temple University.
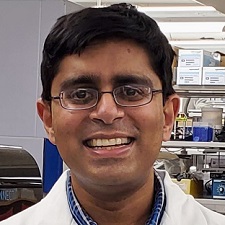
Epidemiologic studies have revealed that many cancer types display differences in incidence or outcomes between the sexes. In most cases, these differences are only partially explained by non-genetic factors such as hormonal differences, carcinogen exposure, lifestyle, and access to health care. Our understanding of how genetic factors contribute to differences in cancer incidence between the sexes remains incomplete. A fundamental genetic difference between the sexes is in chromosome composition. Relative to male somatic cells, female somatic cells have an extra X chromosome. Most genes on the second copy of chromosome X in females are inactivated via a process known as X-chromosome inactivation, which approximately equalizes the dosage of X-linked genes between males and females. Dr. Viswanathan's project tests the hypothesis that genetic alterations to the X chromosome in cancer may perturb this carefully regulated process and thereby contribute to differences in cancer incidence or pathogenic mechanisms between males and females.
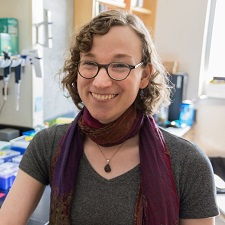
Dr. von Diezmann is a biophysicist who studies how cells regulate the pathway used to repair broken DNA. Errors in specific DNA repair pathways are an early step in the development of many cancers, such as with defects in homologous recombination for breast, ovarian, and pancreatic cancers. The Diezmann lab uses high-resolution microscopy techniques to visualize the process by which DNA breaks are designated for specific repair fates, working primarily in live meiotic nuclei of the model organism C. elegans. By elucidating the mechanisms by which protein assemblies form and transmit information along chromosomes and throughout the nucleus, her lab will help provide a foundation for the development of novel chemotherapies based on modulating the DNA damage response.
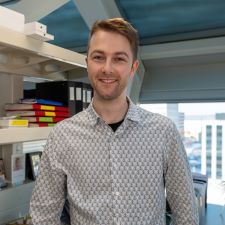
Cancer immunotherapy has revolutionized the way we treat cancer; however, it is only successful in a small subset of patients. Optimally functioning CD8 T cells, the specialized killers of the immune system, are key to the success of cancer immunotherapies. While CD8 T cell function is highly influenced by their metabolism, little is understood about how metabolism changes the function of these cells. Dr. Watson hypothesizes that metabolism affects CD8 T cell function by altering how tightly its DNA is packaged (its epigenetics), leading to altered gene expression. Using a mouse model of adoptive T cell therapy, a widely used immunotherapy in humans, and epigenetic techniques, Dr. Watson proposes to uncover how metabolism influences CD8 T cell epigenetic landscapes to control their function. He plans to apply these findings to improve T cell function and enhance tumor clearance. Dr. Watson received his PhD from the University of Pittsburgh, Pittsburgh and his BS from Hope College, Holland, Michigan.
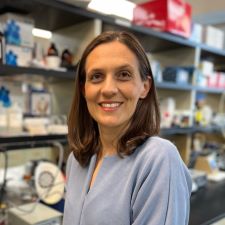
Neuroblastoma is a rare pediatric cancer that typically arises in the adrenal glands, located above the kidney. Children with high-risk neuroblastoma often have poor prognoses despite intense treatment-including maintenance treatment with retinoic acid-underscoring the need for new treatments to improve long-term outcomes. Retinoic acid, which is orally available and generally well tolerated, helps neuroblastoma cells mature (differentiate) into normal cells; however, this process is entirely reversible once the retinoic acid is withdrawn. If this differentiating effect could be made permanent with the addition of a second drug, a combination treatment with retinoic acid could become a novel method of preventing patient relapse. After testing a panel of 452 small molecule drugs, Dr. Weichert-Leahey discovered that a drug called PF-9363 accentuated the effects of retinoic acid in neuroblastoma the most. She will now study how PF-9363 functions, alone and together with retinoic acid, both in cells and patient-derived neuroblastoma models in mice. These experiments will indicate whether combinations of this new compound with retinoic acid may improve outcomes for children with high-risk neuroblastoma.